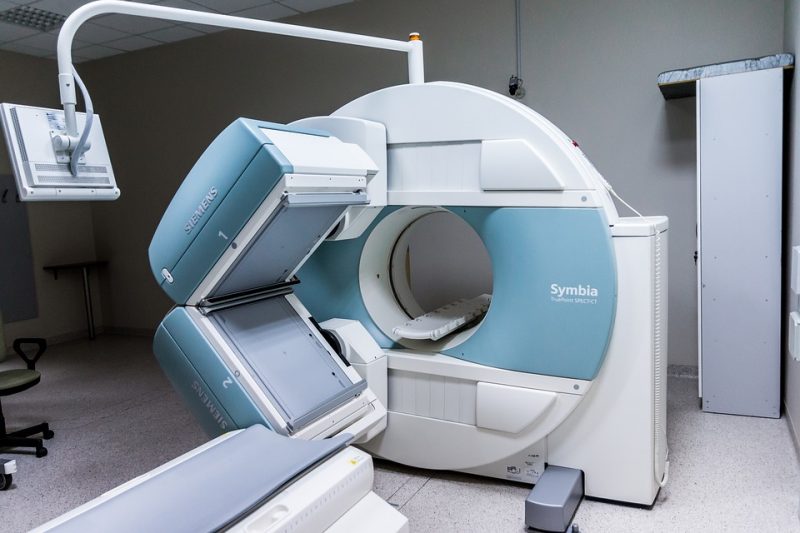
Understanding the brain is one of the challenges of the 21st century and the target of a large number of research projects currently funded around the world. The difficulties are mainly in the complexity of this organ, which occurs at multiple levels (from cells to system).
The brain is made up of cells, including neurons and glial cells, whose size is the order of micrometers (i.e., a thousand time smaller than a millimeter). Studying a single cell is only possible using powerful microscopes, and, for this reason, a great deal of information is derived from animal studies, or from examining slices extracted post-mortem.
The ability to study the human brain directly, in living people, and possibly in patients with a given disease, however, is invaluable. This type of research requires the use of non-invasive techniques to look inside the brain without harm.
Magnetic resonance imaging (MRI) provides such a tool: it can take very detailed pictures of the brain. However, its resolution is limited, meaning that the smallest element in an MRI scan will be of the size of approximately 1mm. How can we tell what is happening at the cellular level? Thanks to the unique properties of MRI, we can.
MRI provides detailed images of the brain, but its resolution is inherently limited. Inside a single pixel, there are millions of cells that we cannot access directly. Image courtesy Mara Cercignani
Why is MRI so special compared to other radiological techniques?
MRI differs from all other radiological measurements, such as CT, because the latter uses an external source of radiation (e.g., x-rays) and then measures the outcome of the interaction between this external radiation source and the human body. By contrast, MRI does not require an external source; it measures a signal that comes from within the body. The MRI signal is produced by the hydrogen contained in water molecules, which make up at least 60% of our body (and brain).
The first consequence of using an internally-generated signal is the exceptional contrast that MRI gives between soft tissues. The second consequence is that the MRI signal carries a large amount of information about the local environment of the water, i.e., about the brain tissue. This means that the MRI signal does not reflect a single tissue property (such as tissue density for CT) but many different properties. And we can produce images of the same brain with many different contrasts by simply tweaking the acquisition. Just like a painting, MRI scans are not made up of a single color but a palette, and the painter can choose how much of each color to use.
MRI can be used to obtain images with different contrast. Each image is weighted to express one component of the signal more than the others do, and therefore yields slightly different information than any other. Image courtesy Mara Cercignani
From conventional to quantitative MRI
Although the versatility of MRI is certainly of great utility in the context of clinical diagnosis, it is not sufficient to provide information about brain cells. A further step is required. So far, we have established that any change to the cell density, tissue orientation, blood supply, etc, will affect the water molecules in tissue and be reflected by the measured MRI signal.
Therefore, we can develop many MRI techniques reflecting differing properties of tissue by making the measurement more or less sensitive to them. For example, we can make MRI sensitive to the microscopic motion of water molecules within the brain. As this motion is affected by the “obstacles” they encounter — represented by cells, membranes, and organelles — it reflects the tissue structure at the microscopic level.
Alternatively, we can make MRI sensitive to the density of proteins and lipids in tissue – thus providing a complementary tool. To extract quantitative information from these images, we need a mathematical model of the MRI signal. In other words, we need an equation that expresses the signal that we measure as a function of the parameters we are interested in (e.g., cell density). Once we have this equation, we can fit it to real data and obtain biologically meaningful parameters from MRI.
The difference between conventional (qualitative) MRI and quantitative MRI: The picture in the left is weighted by the microscopic random motion of water molecules (diffusion), but we can only appreciate difference that can be captured by eye; In the picture in the right, every pixel’s intensity is equal to the diffusion coefficient in that location – this means we can use them as numbers. Image courtesy Mara Cercignani
The value of multi-modal MRI
The sensitivity of MRI to the microscopic environment is unique, but none of the currently available technique is perfect. As the information of interest (e.g., cell density) is derived in a very indirect way (e.g., by measuring the average displacement of water), it is subject to bias. In addition, there is always some degree of overlap between the information captured by two separate MRI techniques. It should not be forgotten that the properties of interest are typically at a scale that is 1000 times smaller than the smallest volume we can measure with MRI. So how can we maximize the information extracted from MRI? MRI scientists are moving toward multi-modal approaches. The idea is to acquire many different MRI techniques of the same brain and, by assessing them all at the same time, extract enhanced information.
The principle is relatively simple, but the implementation is not. In the paper “Brain microstructure by multi-modal MRI: is the whole greater than the sum of its parts”, Cercignani and Bouyagub review differing approaches to multi-modal MRI, that span from “data-driven” approaches, by which the different images are fed into some computational model that can extract salient features, to model-driven approaches, whereby the data are interpreted through biophysical models inspired by the brain composition. Both approaches have advantages and limitations and are subject to the limitations of MRI itself, but this way of re-thinking brain imaging holds a great promise for the future.
These findings are described in the article entitled Brain microstructure by multi-modal MRI: is the whole greater than the sum of its parts, recently published in the journal NeuroImage. This work was conducted by Mara Cercignani and Samira Bouyagoub from Brighton and Sussex Medical School.