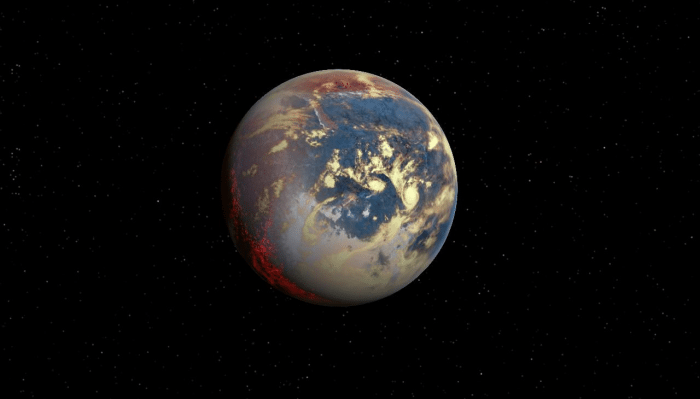
Our knowledge of the Earth’s interior comes from the study of seismic waves during earthquakes, observations of different types of rocks, laboratory experiments, and magnetic and gravitational evidence. But how can we study the interiors of exoplanets, planets orbiting around stars other than our Sun, trillions of kilometers away?
About a thousand super-Earths, exoplanets up to twice the size of our planet, have already been discovered with the help of various space and ground-based telescopes. Iron is considered to be the major constituent of the cores of super-Earths. Numerical models, which are key in understanding the structure of the interior of super-Earths, make use of the equation of state for iron which essentially gives the density of iron at high pressures. The lack of accurate ultra-high-pressure equation of state data for iron forms a bottleneck in this field of research.
Pressures at the centers of super-Earths are extremely high, up to about 100 million times the atmospheric pressure on our planet (10 tera-pascals). Such pressures cannot be created in any laboratory yet. That is when the first principles of quantum mechanics come to our rescue.
In a paper recently published in Icarus, Kaustubh Hakim from the University of Amsterdam and Vrije Universiteit, Attilio Rivoldini and Tim Van Hoolst from the Royal Observatory of Belgium, Stefaan Cottenier and Jan Jaeken from Ghent University and Gerd Steinle-Neumann and Thomas Chust from Universität Bayreut, and implemented the density functional theory to derive a new equation of state of iron valid up to 1370 million times the atmospheric pressure (137 tera-pascals).
Equations of state for iron available in the literature are tailored for pressures inside the Earth. Comparison of this new equation of state for iron with previous equations shows that extrapolations of previous equations beyond their validity range predict the incorrect density of iron by up to 20% at the pressure of 10 tera-pascals. Moreover, the authors show that such inaccuracies lead to erroneous estimations about the interior properties of super-Earths, reinforcing the importance of the use of the newly-computed ultra-high-pressure equation of state of iron.
Further, these authors implement this new equation of state of iron to compute numerical models for interiors of super-Earths. To explore the maximum range of possible compositions, the core is either assumed to be made of pure iron or an iron alloy with 80% the density of pure iron, and the mantle is either assumed to be made of pure MgSiO3 or pure FeSiO3 (pure FeSiO3 is highly unlikely but gives an upper bound on the mantle density). These numerical models are used to produce theoretical mass-radius relations, which make it possible to visually classify observed super-Earths with measured mass and radius.
Based on the relative core size, super-Earths are classified into Moon-like (small core), Earth-like (medium core), Mercury-like (large core), or Bare-core super-Earths. The below figure gives theoretical mass-radius relations and allows to classify super-Earths into different categories. For example, CoRoT-7b can be classified as Earth-like as well as Moon-like, implying that the relative core size of CoRoT-7b is most likely between that of the Moon and the Earth, assuming the atmosphere on CoRoT-7b is thin or non-existent.
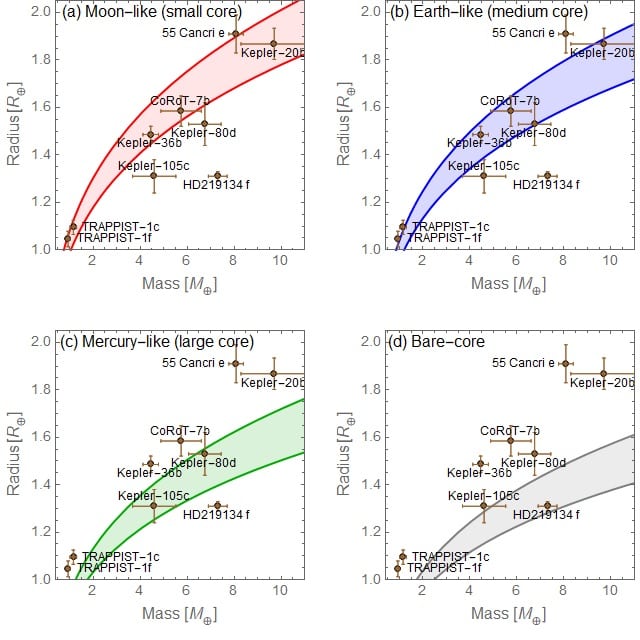
Figure: Theoretical mass-radius relations in the form of shaded color bands for four classes of super-Earths which are based on the relative core size. The extent of shaded bands is determined by the assumption of extreme compositions in the core and the mantle. Observed super-Earths can be categorized into one or multiple classes based on their location in one or more plots. Credit: Kaustubh Hakim
The inability of these numerical models to specifically identify the type of super-Earths from mass-radius relations alone is well-known to astronomers and is known as degeneracy. One of the ways to mitigate this degeneracy is by connecting the numerical models of exoplanets to the composition of their host stars, as shown by Caroline Dorn of ETH Zurich and co-authors in their paper. More research is being conducted in this direction and the future is bright for the explorers of the interiors of super-Earths.
These findings are described in the article entitled A new ab initio equation of state of hcp-Fe and its implication on the interior structure and mass-radius relations of rocky super-Earths, recently published in the journal Icarus. This work was conducted by Kaustubh Hakim from the University of Amsterdam and Vrije Universiteit, Attilio Rivoldini and Tim Van Hoolst from the Royal Observatory of Belgium, Stefaan Cottenier and Jan Jaeken from Ghent University, and Thomas Chust and Gerd Steinle-Neumann from Universität Bayreuth.