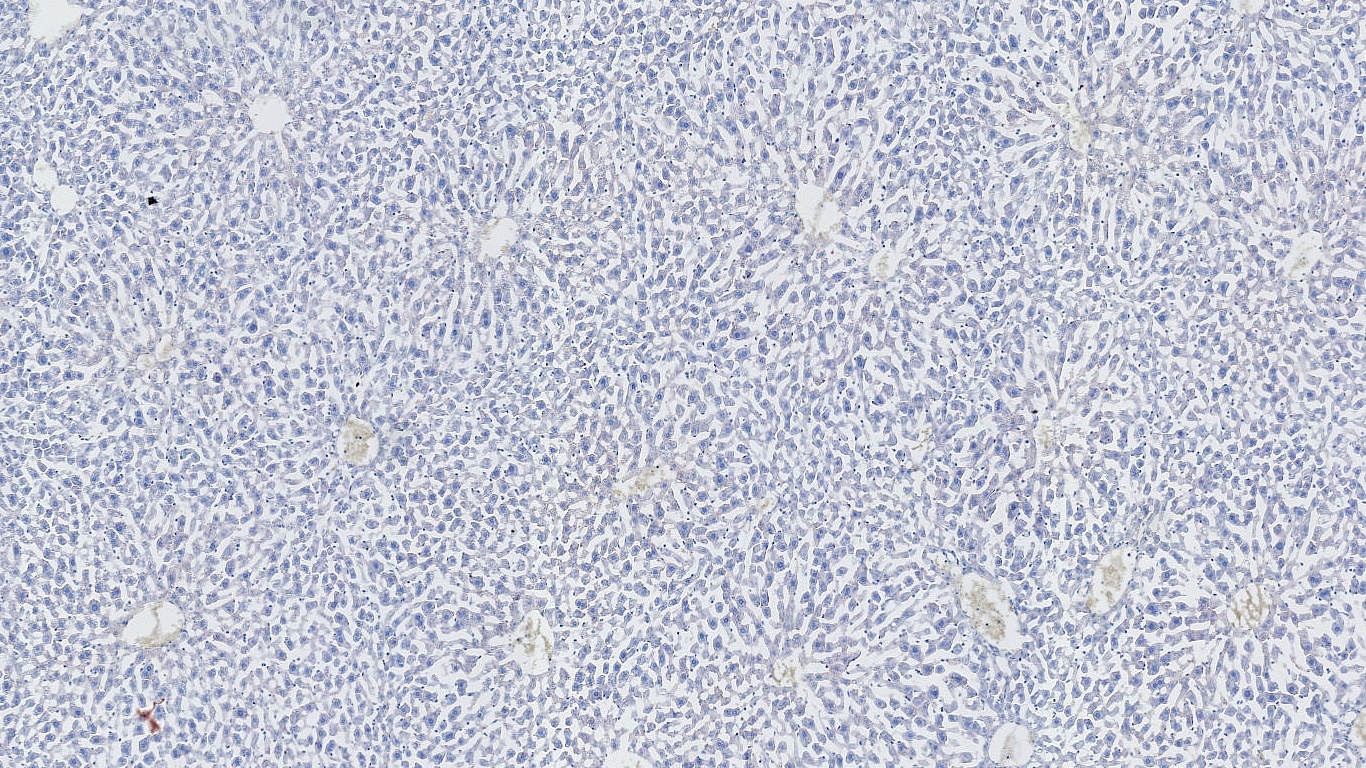
The liver is a vital organ orchestrating initial metabolism of nutrients and xenobiotics after food intake. Moreover, the organ store energy in form of glycogen, which is released in form of glucose when food is scarce. Under prolonged fasting, where the glycogen depot runs low, the liver converts free fatty acids, released from the adipose tissue, to ketone bodies which fuel the brain. Collectively, this puts the liver at the center stage of the transition between fasting and feeding (1).
After a meal, the food passes through the digestive system, and nutrients enter the bloodstream by the portal vein. The portal vein transports nutrients from the digestive system directly to the liver — more specifically into substructural sinusoids lined with hepatocytes, the primary cellular constituent of the liver. The hepatocytes partially metabolize (or store) a large part of the nutrients before they reenter circulation via the central veins of the liver. The central veins drain into the vena cava and eventually into the heart, where the partly metabolized nutrients are pumped via the arteries to the rest of the body.
To control hepatic nutrient and xenobiotic metabolism after food intake, a large number of metabolic processes are tightly controlled. This is achieved by a) regulation of enzymatic activities and b) protein/enzyme synthesis via for example regulation of gene expression (3). I.e expression of genes involved in fasting are downregulated while expression of genes involved in nutrient metabolism and storage are upregulated. We wanted to examine genome-wide gene expression at the transition between fasting and feeding and study molecular mechanisms controlling this expression. Since we needed a tight control over feeding status and accesses to liver tissue, we performed feeding restricted experiments in mice trained to a 12-hour day/night cycle. We allowed mice to feed only during the night (when they normally feed) and isolated livers at the transition between day and night. We subsequently performed a series of genomics experiments to identify genes and regulatory elements of the genome controlled by feeding. Importantly, our study design allowed us to separate feeding regulated gene expression from the core circadian gene regulatory network (4).
Combined bioinformatic analysis of gene expression (also termed gene transcription) and activity of gene regulatory regions of the genome pointed to a possible role of specific transcriptional regulators (or transcription factors) responding to insulin and corticosterone. For example, we mapped binding of transcription factors FOXO1 (negatively regulated by insulin) and GR (positively regulated by corticosteroid) genome-wide and found that these two transcriptions co-occupy the genome in the liver (pre-prandial). Moreover, we found that binding of FOXO1 and GR to the genome is impaired by feeding. Importantly reduced binding correlates with the decreased activity of specific regulatory regions and associated decreased gene expression. We could correlate this with increased circulating insulin levels and decreased corticosterone levels, which gave us an indication that these signaling networks may cooperate to negatively regulate gene expression in the liver in response to feeding.
To experimentally test this, we used a pharmacological strategy to perturb insulin and corticosteroid signaling. Interestingly, we found that these two pathways operate separately (i.e. insulin secretion does not change corticosterone secretion and vice versa) but they cooperate to regulate a major part of the genes suppressed shortly after feeding. To confirm this, we teamed up with Jan Tuckermann at Ulm University and Morris White at Harvard Medical School. This allowed to us genetically perturb insulin and corticosteroid signaling in the liver. These studies agreed with the pharmacological approach and thus illustrates a fine cooperative balance between two hormones secreted out phase during a feeding response.
Interestingly, when we replicated the experiments in diet-induced obese mice, we found that genes regulated by the corticosteroid and insulin signaling were dysregulated in response to feeding correlating with perturbed pre-prandial levels of insulin and corticosterone. Obesity is associated with insulin resistance and perturbed corticosteroid signaling and these studies mechanistically illustrate how this can impact circadian gene expression in the liver.
These findings are described in the article entitled Insulin signaling and reduced glucocorticoid receptor activity attenuate postprandial gene expression in liver, recently published in the journal PLOS Biology.
References:
- Petersen MC, Vatner DF, Shulman GI. Regulation of hepatic glucose metabolism in health and disease. Nat Rev Endocrinol 2017;13(10):572–587.
- McCuskey RS. Morphological mechanisms for regulating blood flow through hepatic sinusoids. Liver Int 2000;20(1):3–7.
- Lin HV, Accili D. Hormonal regulation of hepatic glucose production in health and disease. Cell Metab 2011;14(1):9–19.
- Kalvisa A, Siersbæk MS, Præstholm SM, Christensen LJL, Nielsen R, Stohr O, Vettorazzi S, Tuckermann J, White M, Mandrup S, Grøntved L. Insulin signaling and reduced glucocorticoid receptor activity attenuate postprandial gene expression in liver. PLoS Biol 2018;16(12):e2006249.