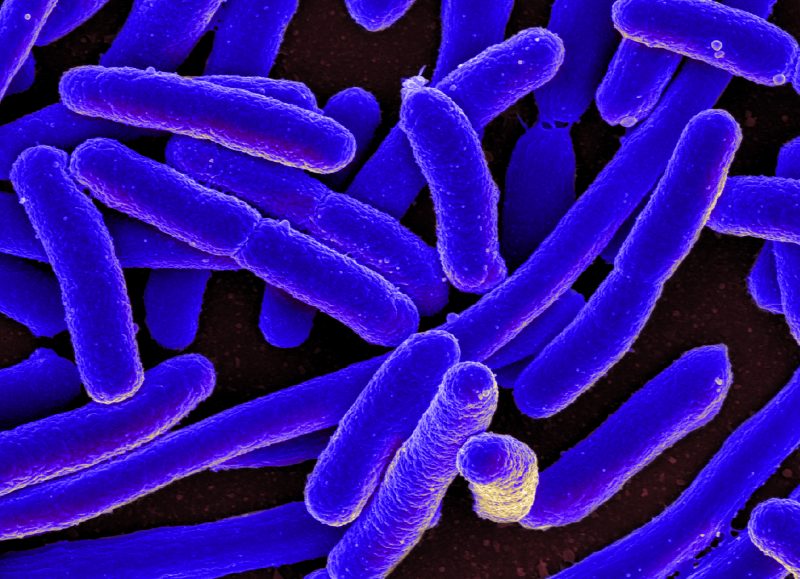
1,000,000,000,000. A trillion. This is the number of species that are estimated to inhabit planet Earth with us.
Organisms of all conceivable sizes, shapes, and lifestyles live on earth. Through the window of my lab in Zurich, Switzerland, I can see a small patch of greenery. It is the end of summer in Switzerland, and the patch is still lush. It hosts a few trees, many other plants, and a few birds. It also hosts many other organisms I cannot distinguish at this distance, and even more that I could not distinguish at any distance, because they are invisible to the naked eye. How did all this diversity arise?
Some of it arose by a process known as “allopatric speciation,” in which a population gets subdivided by a physical barrier that prevents contact between individuals in the two subpopulations. Thus, when mutations arise in each subpopulation, they remain there, and when they accumulate over time, the two subpopulations eventually become so different that they can no longer reproduce with one another – a new species is born. A beautiful example of this process involves snapping shrimp of Central America, where new species arose when the Isthmus of Panama emerged gradually and separated the Caribbean from the Pacific waters of Central America. The similarities between snapping shrimp’s species on the sides of the Isthmus accompany the closure of the Isthmus with more similar species found in shallower waters.
In addition, diversity can arise without any physical barrier through a process called “sympatric” or “ecological” speciation. For instance, when apples were introduced to North America, individuals of the apple maggot flies changed their plant host from Hawthorn to apples. Today, the apple maggot flies population feeds on hawthorns or on apples. This ecological barrier can over time lead to the creation of new fly species, just like a physical barrier would.
These two modes of creating diversity are well-known, but my research focuses on a third fascinating possibility: diversity can arise even when organisms share both the same space and a unique available resource.
At first sight, this may seem impossible. Let’s imagine a population in which all individuals initially use the resource in the same way. When a mutant arises that is inferior at using the resource, it will get eliminated over time. Conversely, a superior mutant will sweep through the population. But it turns out that the two variants – ancestral and mutant – can coexist when the ancestor modifies the environment in specific ways.
Bacteria are microscopic organisms that excrete antibiotics and metabolic waste products, altering the environment in which they live. These environmental changes may have consequences not only in other organisms but also in the very organisms that create them and, under the right circumstances, can result in increased biodiversity. To see how, let’s assume that a population of bacteria can consume a resource that it transforms into an excreted metabolic waste product and that a mutant arises in this population that can consume this waste product. This is not so unusual, as we know many organisms that survive on the waste of others. (Animals, for example, survive on a waste product of photosynthesizing plants – oxygen.) If the mutant can survive on the waste of the original population, it may persist in the population, together with its non-mutated ancestor. Through this process, new diversity can arise.
We wanted to study exactly this process, and for that, we needed to find how easily bacterial populations can produce waste that could help others survive and form stable communities of such waste producers- waste consumers. To do so, we studied what are known as genome-scale metabolic networks. These networks contain all the metabolic biochemical reactions known to take place in a given organism. These metabolic reactions transform resources into the energy required for the cellular processes, allowing organisms to grow and reproduce.
We studied the metabolic network of Escherichia coli, a well-known bacterium that inhabits the intestine of warm-blooded organisms. We simulated the growth of E. coli in 180 different environments which differed in one nutrient and searched for the metabolic waste products that E. coli could not only produce, but that would also allow E. coli to grow when consumed. We found that E. coli could produce approximately 50 waste products in each of the environments tested that could feed E. coli mutants and thus sustain a community of waste producers and consumers. In sum, thousands of communities composed of waste producer and waste consumer organisms could potentially emerge.
An environment offering only one resource can be altered by the activities of the organisms that populate it. All organisms modify their environment. Some do so in a very noticeable way, such as spiders when they create webs or beavers when they build dams. Others do so in more subtle ways, such as plants and earthworms that alter the chemical composition and humidity of the soil. These alterations can create opportunities for mutants that arise in the population and which may coexist with the original population.
A slice of the biodiversity that we observe today may have arisen from a process like the one described here, where the opportunity to increase the diversity is offered by the organisms that inhabit the environment themselves.
These findings are described in the article entitled An enormous potential for niche construction through bacterial cross-feeding in a homogeneous environment, recently published in the journal PLOS Computational Biology. This work was conducted by Magdalena San Roman and Andreas Wagner from the University of Zurich.