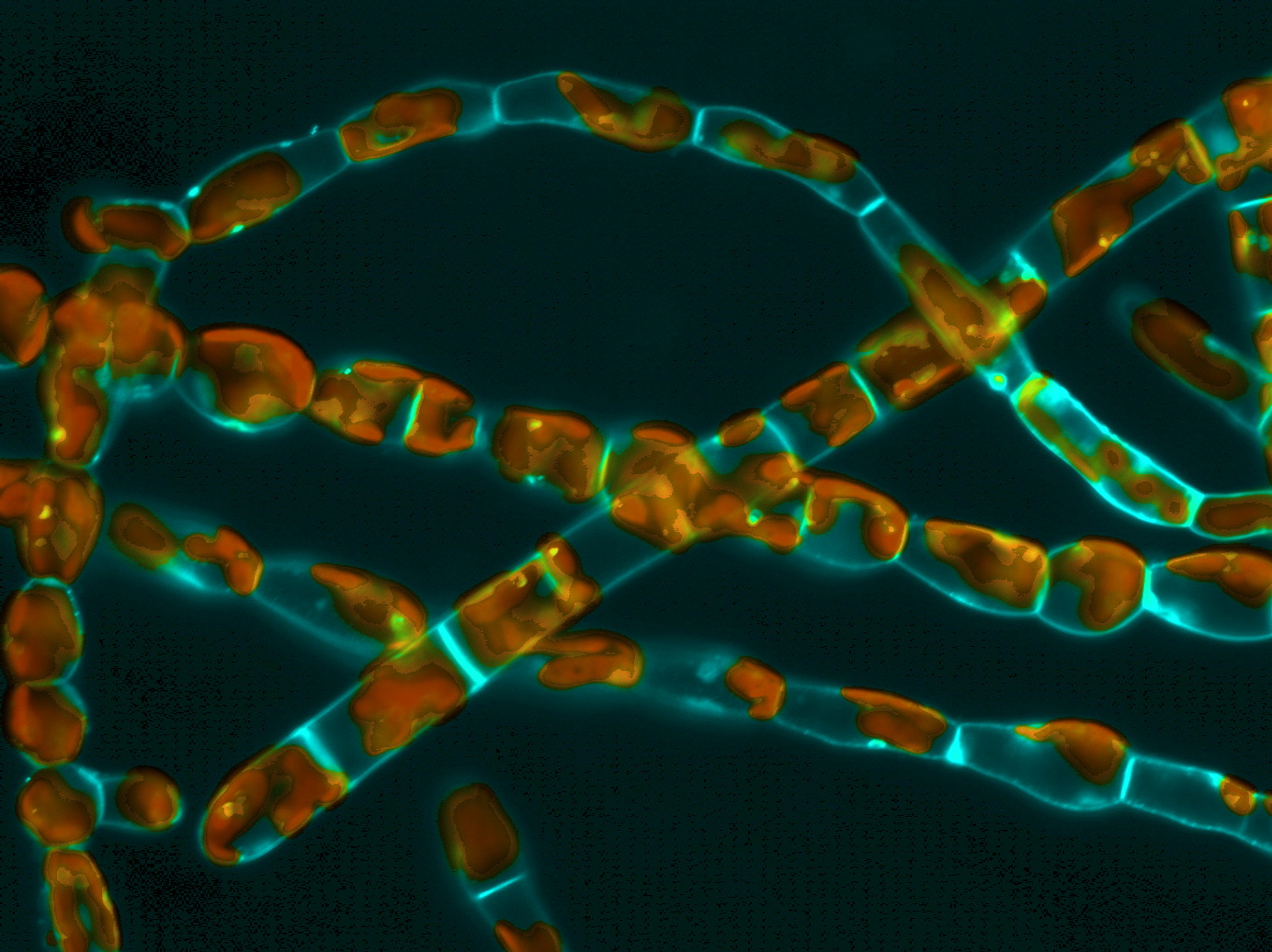
To maintain its shape and grow, a cell needs strength. While animals, whose cells are “bare,” rely mainly on their cytoskeleton, organisms like fungi, plants, and algae are constrained by the presence of an envelope, the cell wall, which can be very rigid. Some of these walled organisms develop filaments made of an aligned cell, among which only the most distal one is responsible for growth: this so-called “apical cell” looks like a closed cylinder with a dome at one end. The dome continuously goes forward, lengthening the cell, which at some point divides, adding one sub-apical cell to the filament.
The engine of this growth, which can be slow (2 μm / hour) or very fast (~ 1 mm / hour) according to the organism, is the turgor pressure, which pushes against the wall with the same intensity everywhere around the cell. The physical laws imply that the more bent the wall of a cylinder, the lower the local stretching tension (stress) is perceived. So, in the case of apical growth, how does the stretch manage to inflate only the curved dome, while the flanks of the cylindrical cell, which are flat, remain unchanged? In plants, this drop in tension in the dome is known to be compensated by a localized softening of the wall. Thus, even if stress is lower at the tip of the apical cell, this is where wall deformation occurs.
Brown algae, although sharing some morphologic and physiologic similarities with green and red algae, evolved on a distinct evolutionary path. They share no parenthood with plants, except the very ancient ancestor, common to all eukaryotes, including animals. Therefore, their development results from a distinct history of random drift and adaptive necessity, making them valuable models for evolution-of-development (evo-devo) studies. By studying apical growth in brown algae, we found that they use an alternative strategy: rather than changing the mechanical properties of their wall, they induce variations in the local stretching tension. Indeed, according to physical laws, the thickness of the wall is also a determining factor in the value of the stretching tension: a tube with a thinner wall will more likely crack under the effect of pressure than a tube with a thicker wall.
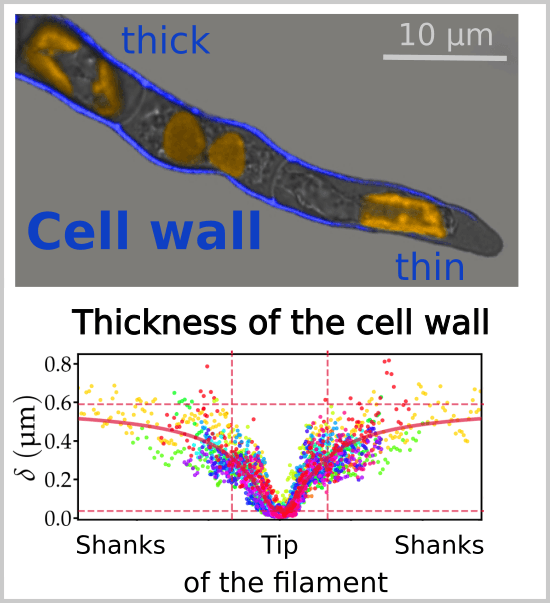
Figure republished with permission from PLOS Biology from https://doi.org/10.1371/journal.pbio.2005258
When we measured the thickness of the wall along the apical filament cells of the brown alga Ectocarpus (from longitudinal sections observed by transmission electron microscopy), we observed that the wall was more than 15 times thinner (36 nm, slightly more than the thickness of a microtubule) at the end of the cell than on the flanks. A simulation using a biophysical model shows that thinning of the wall is exactly tailored to compensate for the decrease in stress due to the curvature of the dome. Under this model, the establishment of a thickness gradient along the cell is critical to both maintain the shape of the cell during growth and control its speed (See animation movie). Thus, by controlling the thickness of their wall, brown algae avoid having to regulate the mechanical properties (viscoplasticity), which would appeal to chemical equilibria and complex enzymatic activities, as in plants.
Thus, the brown alga Ectocarpus, that is subjected to the same physical laws as land plants, reaches the same goal by controlling different key parameters. In the present case, this is the local amount of cell wall materials rather than its chemical composition. This original strategy could be related to the extremely slow growth of Ectocarpus — 2.5 μm / hour, the lowest measured so far — or with its marine environment subject to strong mechanical stresses (currents, waves ) and frequent variations in salinity (tides, rain).
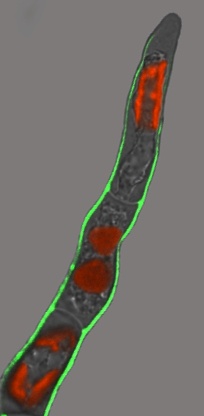
Credit: Author
These findings are described in the article entitled The brown algal mode of tip growth: Keeping stress under control, recently published in the journal PLOS Biology.