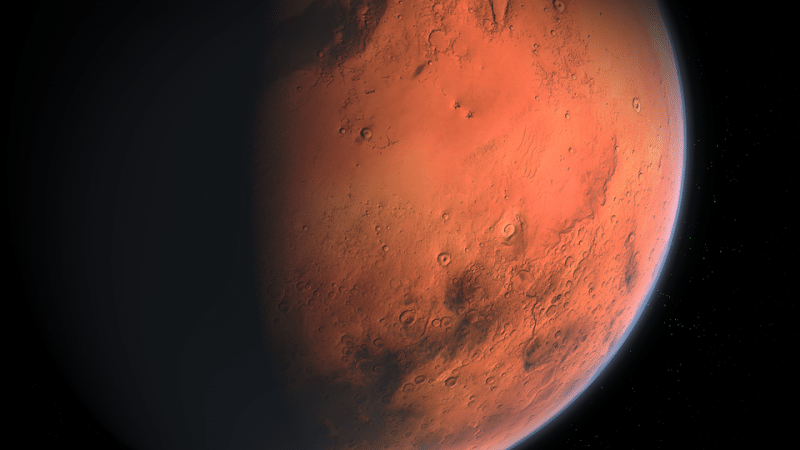
Explaining why Mars is so much smaller and accreted far quicker than the Earth is a long-standing problem in planetary science. Like Earth, Mars’ orbit places it in the Sun’s potentially habitable zone. However, its total mass is only around 10% that of the Earth; too small to still possess the internal heat and magnetic activity to maintain an atmosphere.
What makes Mars so interesting to astrophysicists and planetary geologists is that isotopic analyses of the few meteorites we do have from Mars seem to imply that it formed in just a few million years. Earth, on the other hand, is thought to have taken some tens to hundreds of millions of years to grow to its present size. Because Mars’ primordial building blocks are thought to have been very similar to the Earth’s, some process or event must have shut off its growth after just a few million years. For these reasons, Mars is often referred to as a stranded planetary embryo.
While many competing solar system evolutionary models invoke drastic planetary migration schemes or new modes of accretion to explain Mars’ diminutive size, our study offers a simpler narrative. We begin by hypothesizing that the leading model for explaining the makeup of the outer solar system (Jupiter, Saturn, Uranus, Neptune, their moons, and many icy cometary bodies like Pluto) might also be able to explain Mars’ low mass.
The Nice (as in Nice, France) Model explains many of the outer solar system’s peculiarities by invoking an early planetary instability. This reshuffling of the size and shapes of the giant planets’ orbits (Jupiter, Saturn, Uranus, and Neptune) was originally linked with the Late Heavy Bombardment; a perceived spike in the Moon’s cratering record around 600 million years after the solar system formed. However, better, high-resolution imagery from spacecraft like the Lunar Reconnaissance Orbiter and GRAIL, coupled with improved techniques for dating the Apollo samples have drastically changed our understanding of the Moon’s early history over the last decade. As a result, multiple recent studies have called the existence of the Late Heavy Bombardment in to doubt. Thus, the planetary instability is still the best model we have to explain the structure of the outer solar system, but it could have happened at any time.
Since the Nice Model instability has already been shown in previous studies to be problematic for the survival of the fully formed terrestrial planets (Mercury, Venus, Earth, and Mars), our paper asks whether an instability occurring while the inner planets were still forming might be a successful mechanism for growing a solar system that matches our own. Because their gaseous envelopes imply they formed while gas was still present in the sun’s primordial porto-planetary disk, the giant planets formed much quicker than the smaller terrestrial planets, and can, therefore, have a large effect on the inner planets’ growth.
To study this, we performed nearly 1000 N-body simulations of our proposed scenario using computational resources provided by the University of Oklahoma’s Supercomputing Center for Education and Research (OSCER) and the National Center for Supercomputing Application’s (NCSA) Blue Waters sustained peta-scale computing project. Figure 1 shows a time-lapse example of the evolution of a successful system of planets. When the instability ensues, the proto-Earth and proto-Venus continue to grow, while the Mars analog stops accreting large embryos.
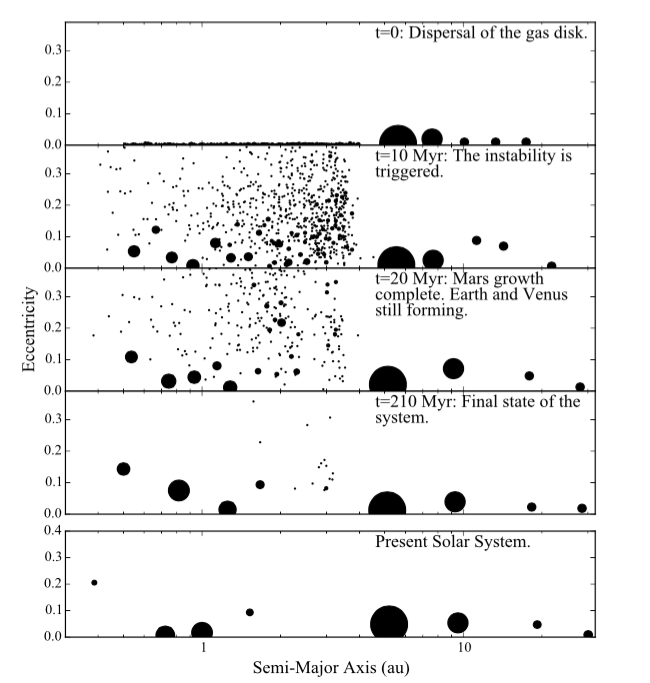
FIGURE CAPTION: Snapshots in time of the growth of a system of planets with a final orientation very similar to the actual solar system (bottom panel). The size of each point corresponds to the mass of the object. The x-axis represents semi-major axis (distance from the sun). The y-axis shows eccentricity (degree to which an orbit is elliptical or non-circular). A video of this simulation can be found at https://youtu.be/2Zm1Hm_JYTY. Republished with permission from Elsevier from: https://doi.org/10.1016/j.icarus.2018.04.008
We found that when we time the giant planet instability just a few million years after the inner planets start to form, “Mars” is just one of several Mars-sized objects in its general vicinity that would eventually combine into a much larger planet if left alone. The result of the instability is that the giant planets’ orbits become increasingly elliptical (non-circular) for a period of time. This increased excitation in the system perturbs the forming terrestrial system as well, particularly the Mars-forming region and the asteroid belt.
This prevents the Mars-sized objects from eventually combining into a larger planet. In fact, most are ejected out of the solar system or displaced inward towards Venus and Earth, often delivering volatile rich-material. In many cases, the system finishes with just one Mars-sized object near Mars’ modern orbit whose accretion was stunted at the time of the instability. Earth and Venus, however, are less perturbed by the giant planets and continue to grow.
Thus, our model naturally explains the disparity between Earth and Mars’ formation times. Furthermore, our study offers a unified and elegant model for the formation of the solar system as a whole. Our simulations are the first to simultaneously reproduce many observed characteristics of both the inner and outer solar system. In fact, the success of the terrestrial planetary formation simulations seems to be largely tied to the detailed evolution of the solar system’s two giant planets: Jupiter and Saturn. Systems in our work where Jupiter and Saturn’s post-instability orbits are most similar to their actual current orbits also produced systems of terrestrial planets that best resembled the current solar system.
These findings are described in the article entitled Mars’ growth stunted by an early giant planet instability, recently published in the journal Icarus. This work was conducted by Matthew S Clement and Nathan A Kaib from the University of Oklahoma, Sean N. Raymond from the University of Bordeaux, France, and Kevin J. Walsh from the Southwest Research Institute.