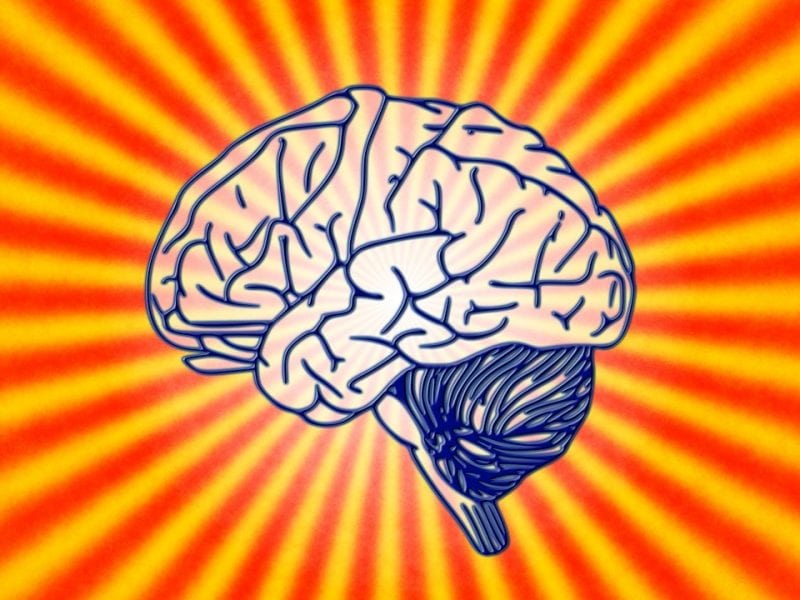
How the human brain controls limb movements is still not fully understood. Many existing models relating brain activity to human movement are derived from animal research, particularly in monkeys. The research in our lab is focused on elucidating how the human brain controls motor behaviors such as arm movements and walking.
Our research utilizes the opportunity provided by epilepsy patients who are undergoing seizure surgery evaluation. In these patients, electrocorticogram (ECoG) electrodes are placed underneath the skull onto the surface of the brain. Normally, these electrodes are used to find the source of seizures so that these abnormal brain areas can potentially be surgically removed to treat the seizure disorder. While these electrodes are implanted for clinical purposes, for us, they present a unique research opportunity to record bioelectric activity from the brain as the patients are instructed to make various arm and leg movements.
Brain Control Of Upper Extremity Movements
How the human primary motor cortex (M1) controls hand and arm movements is not fully understood. Although human ECoG signals are known to change during upper extremity movements, the exact relationship between these signals and the movement trajectories has not been explicitly characterized. To address this issue, we recorded ECoG signals from epilepsy patients as they performed simple movements of the hand, elbow, and shoulder. These movements were systematically varied in speed and duration and were associated with bursts of high-frequency electrical activity in M1.
This high-frequency activity, which resides in the 80–160 Hz range, is known as the γ (gamma) band. The amplitude of the g bursts was directly proportional to the movement speed. In other words, faster movements were associated with more powerful gamma bursts. We also found that higher movement speeds involved activation of larger M1 areas. Likewise, the duration of g bursts increased proportionately with movement duration. In summary, upper extremity movement speed appears to have a direct relationship with M1 activation intensity, duration, and area – suggesting that the neurons in M1 may have direct control over upper extremity muscles.
Brain Control Of Lower Extremity Movements
Compared to arm movements, even less is known about how the human M1 controls walking. By recording ECoG signals from M1 as the patients walked on a treadmill, g bursts occurred during walking and they were rhythmically locked to each stride. The same pattern was observed even when patients walked at different speeds. We also found that the M1 areas associated with walking were not the same as those responsible for movement at individual joints of the leg. These observations suggest that M1 uses different strategies to control walking and individual joint movements of the leg. One plausible explanation is that M1 primarily provides macro-level control of walking, such as the decision to initiate or cease walking, and speed up or slow down.
Meanwhile, micro-level control such as generating the leg muscle activation patterns or leg movement trajectories are provided by the neuronal networks within the spinal cord. In other words, the role of M1 in human walking could be to provide instructions to these spinal networks, which are then responsible for generating the muscle activity that produces human walking.
What Can This Information Be Used For?
Not only do these findings deepen our understanding of how the brain controls different movements, but they can also be applied toward the development of neural technologies. For example, this knowledge can be leveraged for brain-computer interfaces (BCIs) – a technology which translates brain bioelectrical signals into control commands for external electromechanical systems.
BCIs have the potential to help people with paralysis due to spinal cord injury or stroke regain movement, by connecting their brains to prostheses, thereby bypassing the site of injury.
References
- Wang PT, McCrimmon CM, King CE, Shaw SJ, Millett DE, Gong H, Chui LA, Liu CY, Nenadic Z, Do AH. Characterization of electrocorticogram high-gamma signal in response to varying upper extremity movement velocity. Brain Structure and Function. 2017 May 18:1-44.
- McCrimmon CM, Wang PT, Heydari P, Nguyen A, Shaw SJ, Gong H, Chui LA, Liu CY, Nenadic Z, Do AH. Electrocorticographic encoding of human gait in the leg primary motor cortex. Cerebral Cortex. 2017 Jul 11:1-1.
- King CE, Wang PT, McCrimmon CM, Chou CC, Do AH, Nenadic Z. The feasibility of a brain-computer interface functional electrical stimulation system for the restoration of overground walking after paraplegia. Journal of neuroengineering and rehabilitation. 2015 Dec 1;12(1):80.
These findings are described in the articles entitled Characterization of electrocorticogram high-gamma signal in response to varying upper extremity movement velocity and Electrocorticographic Encoding of Human Gait in the Leg Primary Motor Cortex, published in the journals Brain Structure and Function and Cerebral Cortex, respectively. This work was led by An H. Do & Zoran Nenadic from the University of California, Irvine.