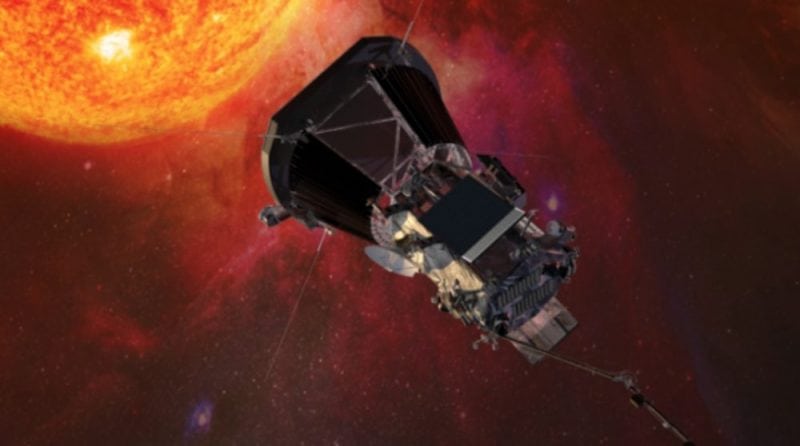
Parker in ApJ 1958 developed the supersonic solar wind theory and predicted the known today spiral shape of the solar magnetic field. His theory on the supersonic expansion of the solar wind was verified observationally a few years afterward in the 1960’s. Since Parker’s paper, a plethora of space and ground-based telescopes have been operating providing continuous observations of the solar wind plasma and its variations in different longitudes and latitudes. Nevertheless, until the launch of Parker Solar Probe by NASA later this year, i.e. 60 years from Parker’s initial paper, there has not yet been any telescope that takes in-situ measurements close to the Sun.
Parker Solar Probe will be the first spacecraft to approach the Sun in such proximity, diving into the very region where the solar wind originates from, providing us with in-situ measurements of quantities, such as density, temperature and ion abundances and valuable insights on processes, such as the corona heating.
Models
For all those years, scientists had to rely on models to propagate the assumed or observationally inferred close-to-Sun conditions to match the in-situ observations in larger heliocentric distances. In this study, we are using two models with assumptions that lie at the two opposite ends of the collisionality spectrum, i.e. a fully collisional single fluid Magnetohydrodynamic (MHD) and a collisionless kinetic exospheric model.
Single Fluid Model
On the one hand, the single fluid model is data-driven, a) using a photospheric magnetogram as input, b) performing a Potential Field Source Surface (PFSS) extrapolation, c) using semi-empirical models and physical arguments of energy and momentum conservation to calculate the three-velocity (v), temperature (T) and density (ρ) from the three-magnetic field (B), and finally d) using all eight MHD quantities to run an MHD simulation from 0.1 astronomical unit (AU) to 2AU. The acceleration of the solar wind in the fluid model is due to the injection of energy in the system in the form of heating. The MHD model is a robust 3D model capturing the evolution of the 3D solar wind variability. The heat flux prescribed in the fluid model is not due to a self-consistent mechanism, but rather a convenient formulation to account for the acceleration of the outflow up to the supersonic regime observed at larger distances.
Kinetic Model
On the other hand, the kinetic model we are using is a fully collisionless model of the solar wind. We assume two particle populations, i.e. electrons and protons, which are about 90% of the solar wind particles. All the physical information in the kinetic model exists in the Velocity Distribution Function (VDF) assumed for each population. We make the observationally inspired choice of a Kappa VDF for the electrons, with heavy non-thermal tails and a Maxwellian VDF for the protons. An induced electric field is the one self-consistently accelerating the solar wind while enforcing the quasi-neutrality and zero current conditions at all times similarly to the fluid model. The kinetic model used here was initially 1D, but we parallelized it and generalized it to solve the 1D problem on a spherical grid, essentially allowing for 3D studies.
Analysis And Results
We interface the two models at the inner MHD boundary, i.e. at 0.1AU and after we evolve both of them up to 2AU, we compare their energetics with observations at the orbit of the Earth (1AU) and at the orbit of Ulysses at about 1.4AU during August 2007. When compared to satellite observations at large distances both models do fairly well, being able to reproduce the number of peaks at similar times as observed in the velocity and density profiles, with the MHD model being able to capture more accurately the peaks’ sizes as well. The reason for this is the fact that the kinetic model has a more efficient acceleration mechanism and the initial conditions at the inner boundary were tailored for the MHD model to reproduce results close to the observed values at 1AU, given its specific acceleration mechanism.
The MHD model was able to capture the temperature observed at large heliocentric distances in order of magnitude, while the proton temperature was one order of magnitude smaller and the electron temperature one order of magnitude larger than the observed value at all times. The temperature variability was not captured accurately by any model. The heat fluxes for both models are roughly in agreement with recent observational studies. The electron heat flux is larger than the proton heat flux by an order of magnitude at all heliocentric distances. The MHD heat flux is similar to the electron heat flux profile close to the Sun, but it drops faster in larger distances approaching the proton heat flux profile.
Summary And Conclusions
We used two very different in nature models, essentially lying in the two sides of the collisionality spectrum, i.e. a collisional fluid model and a collisionless kinetic model, for a first meaningful comparison of their energetics. The acceleration in the fluid model was due to heating injected into the system, while in the kinetic model an induced electric field is the one accelerating the outflow to supersonic speeds. Both models did reasonably well when compared to observations, with a few differences due to their different acceleration mechanisms.
A self-consistent hybrid kinetic-MHD model can be developed taking advantage of both the robust 3D description of the MHD model and its data-driven nature in combination with the non-thermal VDFs and the self-consistent heat fluxes of the kinetic model for a more physical description of the solar wind.
These findings are described in the article entitled Interfacing MHD Single Fluid and Kinetic Exospheric Solar Wind Models and Comparing Their Energetics, published in the journal Solar Physics. This work was led by Sofia-Paraskevi Moschou from the Harvard-Smithsonian Center for Astrophysics.