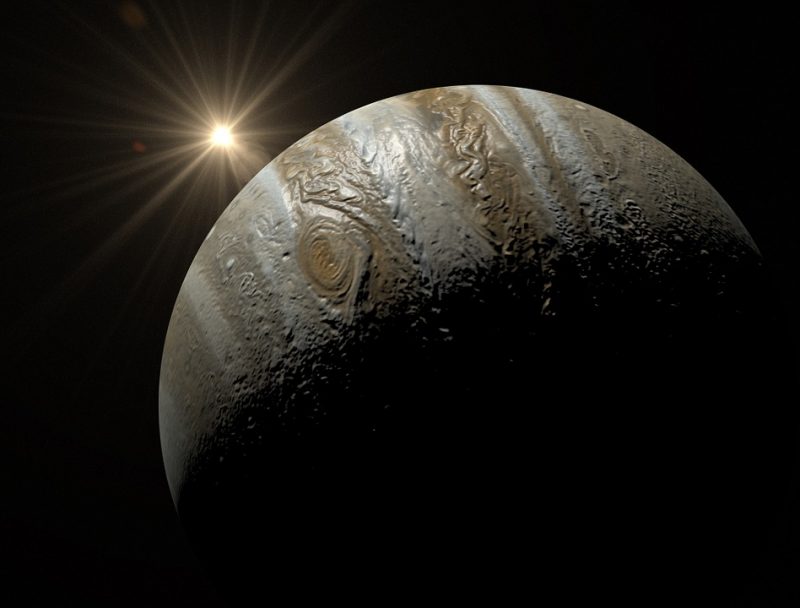
Understanding the shrouded interior of Jupiter is a holy grail of planetary science and astrophysics. Jupiter has more than twice as much mass as all the other planets, moons, asteroids and comets all combined. Everything in the solar system is believed to have coalesced from the same disk of material shortly after the formation of the sun. So in order to understand how the Earth and the rest of the solar system formed, we need to understand what that disk was made out of and how it behaved. There is no larger sample of that primordial disk than the interior of Jupiter itself.
The importance of Jupiter and its interior has not been lost on the scientific community; NASA has sent nine spacecraft to Jupiter, most recently sending the billion dollar Juno mission in 2016 dedicated to understanding Jupiter’s interior. So far, the tools at our disposal have been to measure gravity, magnetic fields, and different wavelengths of light. These tools are powerful and indispensable, but none of them can fully characterize Jupiter’s interior — they can only test different theoretical models for its interior structure.
Seismology revolutionized our understanding of the interior of Earth, and later of the sun. Using Saturn’s rings as a seismograph, scientists have recently begun to detect seismic activity in Saturn as well. Most recently, a team from the Nice Observatory now thinks they’ve detected seismic waves on Jupiter using an Earth-based telescope [1]. This discovery could herald a fundamental paradigm shift in our understanding of Jupiter’s interior, and maybe other planets in the solar system too.
The catch is, current theoretical understanding of Jovian seismic excitation sources (which we give the pithy although somewhat inaccurate designation: “Jupiter quakes”) cannot explain how Jupiter’s seismic signature is so large. In our work, “Excitation Mechanisms for Jovian Seismic Modes,” we try to come up with and simply model realistic “Jupiter quakes” to see if they can produce the observed signal.
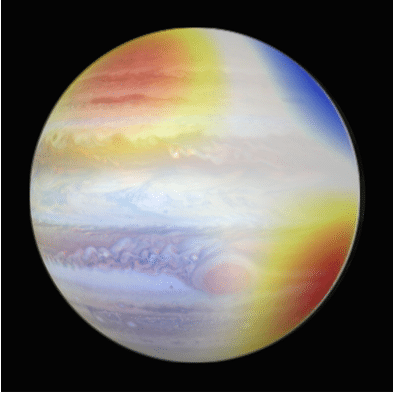
Figure 1: Schematic of an l=2 normal mode superimposed on Jupiter. Red regions represent outward displacement, blue represent inward. SYMPA can measure the Doppler shift this causes and make a velocity map to infer the frequency of the normal mode. Credit: Steve Markham
We test meteor strikes (which create waves in Earth’s atmosphere, and seismic signals in the Earth’s crust) [2], turbulent convection (which creates seismic signals in the sun) [3], and storms. We find that the only way to produce the observed signal out of the mechanisms we considered is with storms that are individually much more energetic than we expect water based thunderstorms to be.
One candidate we identify as a possibility is rock storms, which may exist as enormous storm complexes nearly a thousand miles below Jupiter’s surface. These storms could rocket hundreds of miles upward while raining globs of magma—and just might be energetic enough to make the whole planet tremor.
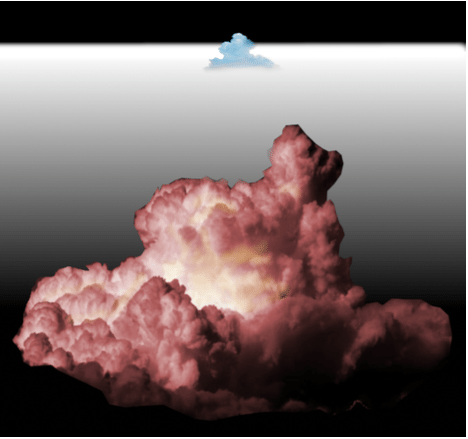
Figure 2: Cartoon showing the approximate expected length scales associated with water storms (blue, top) and rock storms (red, bottom). Credit: Steve Markham
There may be other more familiar excitation sources which have not been considered in this pilot paper. For example, so-called “baroclinic instabilities” are known to develop in Jupiter’s atmosphere [4] due to the difference in sunlight intensity between the equator and the poles. These instabilities produce large amounts of turbulence, which may couple to Jupiter’s normal modes (the quanta of Jupiter’s seismic activity) strongly enough to create a seismic signature. Investigating this possibility, as well as modeling the interaction between storms and seismic modes in more detail, are subjects of future and ongoing research.
If we can form a more complete picture of the excitation sources for seismicity in giant planets, we may be able to make the theory predictive, so that we can guess how seismically active a planet should be. This has important applications for future spacecraft missions. The interiors of Uranus and Neptune are currently not at all understood, and NASA has designated one of these two planets to be targets for a flagship class spacecraft mission in the coming decades. Four of the five proposed missions include room for the tools necessary to do seismology from orbit. If we can predict how seismically active we expect these planets to be, this could provide needed calibration information for these instruments, which could potentially have multi-billion dollar consequences for the success of the mission.
Seismology was the single greatest scientific and technological advancement for our understanding of the interior of the Earth. We identified the Earth’s liquid iron core, its solid inner core, and a much of the detailed structure of the Earth’s mantle using this technique. Helioseismology, studying the frequencies of normal modes in the sun to infer details about its internal structure and behavior, likewise advanced our understanding of the sun’s interior, unmasking its differential rotation, and its deep radiative-convective boundary. In both of these cases, we understand where the excitation sources come from: earthquakes on the Earth, and the large-scale roiling on the sun, as its heat tries to escape from the interior to the surface. We are now just at the beginning of applying those techniques to planets like Jupiter, and we are likely to learn much more about both Jupiter’s interior and its mysterious “Jupiter quakes” as this field continues to develop.
These findings are described in the article entitled Excitation mechanisms for Jovian seismic modes, recently published in the journal Icarus. This work was conducted by Steve Markham and Dave Stevenson from the California Institute of Technology.
References:
- P. Gaulme et al., Detection of Jovian seismic waves: a new probe of its interior structure. Astronomy & Astrophysics, 531 A104 (2011)
- W.N. Edwards et al., Seismic observations of meteors: coupling theory and observations. AGU Publications (2008)
- P. Goldreich et al., Excitation of solar p-modes. Astrophysical Journal, 424 (1). pp. 466-479 (1994)
- P.J. Gierasch et al., Baroclinic instabilities in Jupiter’s zonal flow. Icarus 40, 2 (1979)