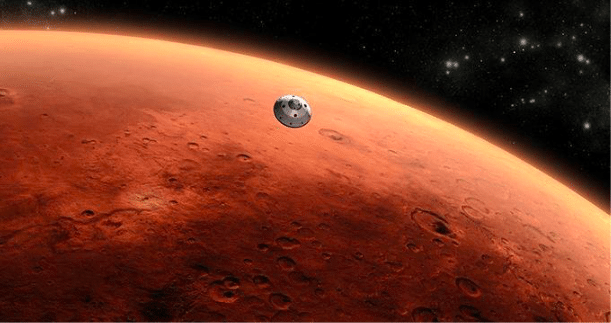
For the Mars surface exploration mission, a vehicle, e.g. Mars Science Laboratory (MSL), should fly through the atmosphere and land on the Mars surface using the deceleration systems such as parachutes and retrorockets. The entry vehicle needs to make use of the aerodynamic force for removing nearly 99% of its kinetic energy before landing on the target point.
However, the thin and highly uncertain atmosphere, the errors of entry states and the vehicle design deviation pose challenges to the entry process.
Recent Mars entry vehicles are designed to have the ability of lift control, so the entry trajectory can be modified by controlling the aerodynamic force. The assessment of the potentially reachable footprint under uncertainties of atmosphere, entry states, and vehicle design will be helpful for Mars mission designers to decide whether the predetermined targeting point needs to be updated. The footprint composed of the longitude and latitude states of the vehicle is a subset of the so-called reachable set depicting the position and the velocity of the vehicle (see figure 1).
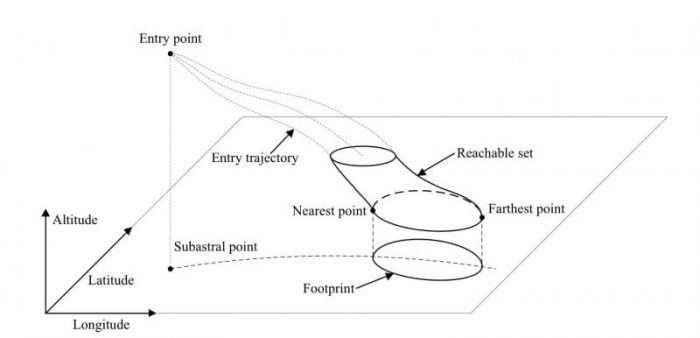
Figure 1: Diagram of the reachable set. Republished with permission from Elsevier from https://doi.org/10.1016/j.asr.2017.09.028
The MSL is the latest vehicle model in the successful Mars entry missions so far. A specific entry mission for the MSL is simulated, in which the vehicle enters the Mars atmosphere with the altitude of 124 km, the longitude of -90 degree, the latitude of -44 degree, the velocity of 5.5 km/s, the flight path angle (FPA) of -13 degrees and the heading angle of 84 degree.
The complete footprint is difficult to generate due to its resulting intensive computation. Therefore, we make some computational assumptions for computation efficiency but without loss of the essence of the problem. The footprint under nominal conditions, in which the uncertainty is not considered, is shown in figure 2. That is to say, the vehicle can theoretically reach the region in figure 2 within its control ability safely. However, it can be found from the following results that the uncertainties existing in the entry dynamic parameters as mentioned before have great effects on the footprint.
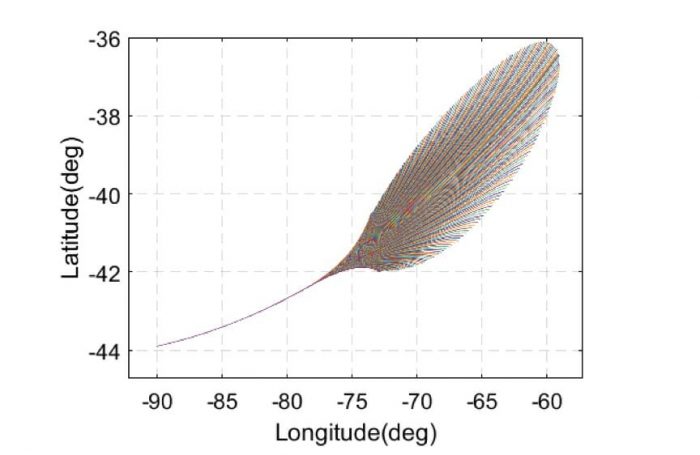
Figure 2: Nominal footprint without entry uncertainty. Republished with permission from Elsevier from https://doi.org/10.1016/j.asr.2017.09.028
Although those uncertainties are not known precisely, their statistic characteristics can be obtained by experimental and measured data. The propagation of the footprint under uniformly distributed uncertainties in initial FPA, lift-to-drag ratio and atmospheric density is shown in figure 3. The uncertainty levels are set as 0.2 degrees in initial FPA, 25% in lift-to-drag ratio and 20% in atmospheric density. The footprint varies from the minimum area in dark blue to the maximum area in bright yellow.
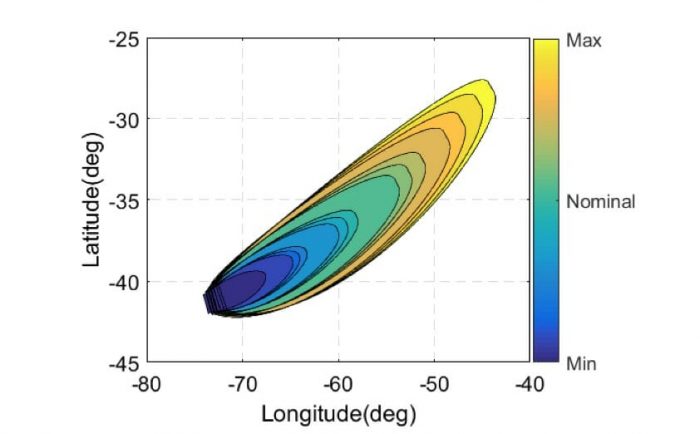
Figure 3: Footprint propagation under uncertainties. Republished with permission from Elsevier from https://doi.org/10.1016/j.asr.2017.09.028
The downrange length of the footprint ranges from about 301 km to nearly 1563 km with consideration of the uncertainties. For comparison, the nominal downrange length is 709 km. Therefore, if the targeting point of Mars mission is set far from the center of the footprint, the predetermined site may be unreachable under the influence of the uncertainties considered above. Besides, the farther the targeting point is from the center of the footprint, the more sensitive it is to the uncertainties.
Furthermore, the uncertainties should also be considered in the safety design of the vehicle, such as the g-load and the thermal protection. The actual g-load, heating rate and total heat of the entry vehicle usually vary and maybe exceed the predesigned limits due to the existence of uncertainties. So the mission design of entry process should be more conservative with consideration of the stricter constraints.
These findings are described in the article entitled Uncertainty analysis of reachable set for planetary entry using polynomial chaos, published in the journal Advances in Space Research. This work was led by Yuechen Huang, Ph.D., Haiyang Li, Ph.D., and Jin Zhang, Ph.D., from National University of Defense Technology, China.