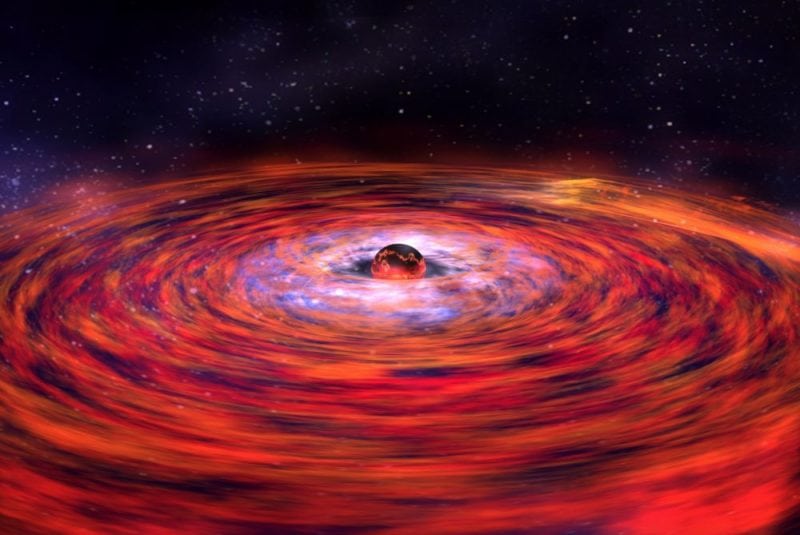
General Relativity is, currently, the best theory to explain gravitational phenomena. Published by Albert Einstein in 1915, the theory found agreement with known astronomical observations, and it stated novel predictions that have been confirmed with great accuracy. Such predictions are actually groundbreaking: the bending of light, the existence of gravitational waves, black holes (yet to be directly confirmed), among others. The genius of Einstein cannot be overstated.
However, the theory is not perfect. On philosophical grounds, none mathematical representation of reality is perfect. But also physically, General Relativity fails to explain galaxy rotation curves, galactic bending of light, and cosmological data. Such discrepancies between the theory and reality can be solved if we assume the existence of the so-called dark matter. Most physics seem to rely on this hypothesis, but it has a serious drawback: although huge efforts, dark matter has not been directly detected.
In this context, it is at least reasonable to think of other ways to solve the problem. If we are not willing to assume the existence of dark matter, then we are forced to modify the laws by which gravity works, i.e. to look for a new theory of gravitation. This is a long enterprise that may be traced back to 1983, with the work of Mordehai Migrom and his MOND theory. Since then, several modified gravity theories have been proposed, and several have stayed behind.
One of the latest is Scalar-Tensor-Vector Gravity (STVG) theory, also referred as Modified Gravity (MOG). Published by John Moffat in 2005, the weak field limit of this theory predicts an enhanced gravitational attraction on the boundaries of galaxies, where phenomena related to dark matter use to happen and agrees with General Relativity inward. The behavior of this weak field limit served, for instance, to correctly describe galaxy rotation curves, galactic light bending, and the Bullet Cluster phenomena, without requiring the existence of dark matter.
The transition of a standard to enhanced gravitational attraction comes from the interplay between tensor, vector and scalar physical fields. However, this mechanism seems to work solely where gravity is weak. Close to black holes or other compact objects like neutron stars, the gravitational field is very strong and Moffat’s mechanism to retrieve General Relativity breaks.
Then, General Relativity and STVG also differ at the strong gravity regime. Such physical systems are key to compare both theories, and our work focuses on them: we found, for instance, that the proposed vector field leads to bigger and heavier neutron stars than General Relativity. On the other hand, the vector field would play a crucial role in the formation, collimation, and rotation of astrophysical jets, ejected by active galactic nuclei. Further, accretion disks around STVG black holes turn to be colder and underluminous than in the case of GR.
While we keep exploring the discrepancies between both theories, we anxiously expect the outcomes of strong gravity observations, headed by gravitational waves and the Event Horizon Telescope. They will have the last saying in this discussion.
These findings are described in the article entitled Effects of Scalar-Tensor-Vector Gravity on relativistic jets, published in the journal Astrophysics and Space Science. This work was led by Federico G. Lopez Armengol from the Instituto Argentino de Radioastronomía.